Emilio MORALES; Marissa CAMACLANG; Gilberto REYES
Chairman, PICE Geotechnical Committee; Member, PICE Geotechnical Committee; Member, PICE Geotechnical Committee;
The tragic loss of 58 lives in the Cherry Hills landslide attracted a lot of Philippine & International media attention and with it a lot of speculations and rumors as to what caused the slide. Several professional organizations and government agencies were also involved in the various studies and investigations to understand what really happened.
This report summarizes and documents the work of the committee, including the methodologies and tools employed in reconstructing the conditions before and after the landslide, its findings and conclusions.
This paper summarizes the collective independent findings and conclusions of the authors.
I. INTRODUCTION
Right after the Cherry Hills Landslide, which occurred on the night of 3 August 1999, the Philippine Institute of Civil Engineers (PICE) and Association of Structural Engineer of the Philippines (ASEP) mobilized members of its Geotechnical Committees in order to volunteer its services in the documentation of the Cherry Hills Landslide. The intention was to focus its attention on the Engineering aspects of the overall investigation. It was necessary to understand what caused the landslide, how the landslides occurred and how such mechanism/s can be prevented or avoided in the future. It is the intention to disseminate this report to all interested parties and to the Engineering Profession in particular so that lessons learned can be applied to prevent future similar accidents.
2. BACKGROUND OF CHERRY HILLS LANDSLIDE
(1) Location of Cherry Hills Subdivision
The subdivision is located in Antipolo City, Province of Rizal, Luzon Island, the Philippines. It is situated in saddle like fashion in an elevated terrain cut from a mountain leaving the East and West Flanks.
It is flanked by two subdivisions, Park Village Subdivision to the North and Peace Village to the South. The East and West flanks are bounded by the mountain sides left after the cut. The Eastern Summit is at elevation 255m Map and the Western side is at elevation 233m. The subdivision cut from the mountain side is approximately 26m at its deepest.
(2) Extent of Development of Cherry Hills
The subdivision encompasses an area of approximately 5 Hectares. All the roads have been paved with plain Portland cement concrete.
The subdivision residential units consist of two and single storey houses that are either single detached or row housing. These are light roofed houses with hollow block wall construction and reinforced concrete columns on isolated footings.
(3) The Landslide
The landslide occurred at approximately 7:00 PM of August 3, 1999. Several hours before the event a lot of residents have evacuated their homes because of telltale signs of the impending slide. Cracks were being observed in some houses which continued progressively, thus, providing ominous signs that were heeded by some.
When the landslide occurred, it was very sudden and according to some eyewitnesses, occurred within five seconds. Two loud noises were heard during the landslide. Fifty Eight (58) lives were lost and one unaccounted for in this slide event.
Interviews with some homeowners indicated that there have been manifestations of movements in the Subdivision platform (creep) as manifested by crackings on the walls and difficulties encountered in the closing and opening of doors and windows. this happened several months before the landslide.
3.0 DATA GATHERING FOR THE ENGINEERING STUDIES
With the data already collected on the Geology of the area and specifically the orientations of bedding planes and joints in the rock mass, the Geotechnical Investigation Team concentrated on the following objectives:
(1) Data Gathering
a) Reconstruction of the Digital Terrain Model (DTM) of the area from the National Mapping and Resource Information Agency (NAMRIA). Maps were done as the reference point for the subsequent subdivision development, to establish platform elevations.
b) Mapping of the slide zone and Cherry Hills Subdivision in relation to the existing Digital Terrain Model by Global Positioning System (GPS) Surveys.
c) Gathering of subsurface data by conducting soil exploration in the slide zone and the undisturbed areas.
d) Gathering of intact block samples for strength testing of cores obtained and classification of soil samples using the Unified Soil Classification System (USCS).
e) Extraction of rock cores from intact blocks and simulation of the effects of groundwater conditions on intact cores through saturation (obtained from within the same sample block).
(2) Analysis of Strength Data
Numerous Unconfined Compression Tests (UCT) were conducted on intact cores obtained from borings and from corings done on block samples gathered from the slide areas. The cores consisted of the various layers of sandstone and the thin siltstone seams interbedded with the sandstones. The results of the Unconfined Compression Strength Test show the range of values of Uniaxial Compressive Strength (qu) of the rock layers in the area.
The Scatter Chart in Figure 1.0 below shows the values for both rock types obtained from the borings.
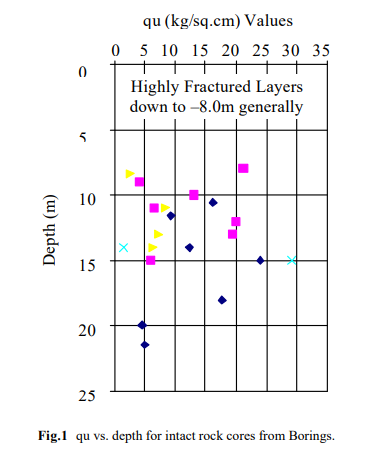
Although these represent the material strengths of the intact rocks from a generic sense, it does not give an idea as to the actual Characteristic Rock Mass Strength (CRMS) during the slide. The Characteristic Rock Mass Strength is the “average” strength of the rocks along the failure plane at failure.
For determining the latter, it would be necessary to consider the degree of jointing, weathering and the other environmental factors that could affect the characteristic strength.
The Uniaxial Compressive Strengths from intact cores served only as the reference base or starting point from which approximations of the CRMS at impending slide could be obtained. This will be discussed subsequently.
(3) Effect of Water Saturation on Strength of Cores
The cores from within the same block samples were grouped and subjected to immersion for 24 hours, 48 hours and 72 hours.
The plot of the Unconfined Compressive Strength qu (kg/cm2) vs. Moisture Content (%) is presented in Figure 2.0 for Sandstone and Siltstone Cores.
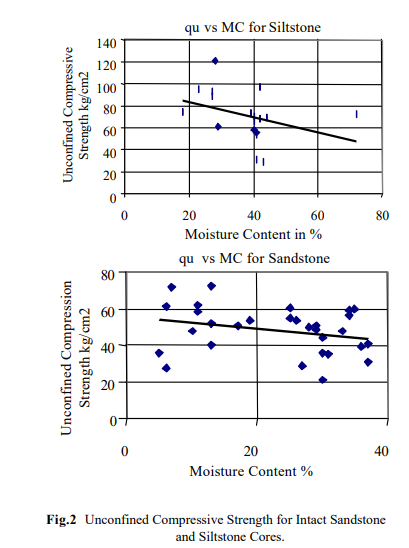
The trendline indicate the decrease in qu with increasing moisture content clearly establishing that water saturation could have an effect on the strengths.
(4) Climatic Effects
The unusually wet weather and abnormally high rainfall occurred in the months before the landslide. This was preceded by the El Niño which in contrast is characterized by extended droughts and unusually very dry and hot climate.
This phenomenon was followed by abnormally high rainfalls in the months and days immediately preceding the slide. (Fig. 3.0)
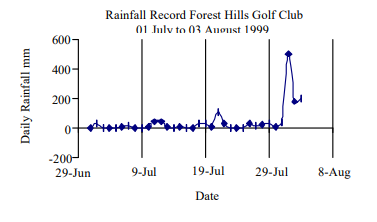
(5) Test for Expansion Potential
The siltstone materials were pulverized and tested based on the Unified Soil Classification System (USCS).
All the siltstone materials classified as “MH” (Highly Plastic Silt). The very high Atterberg Limits. Liquid Limit (LL) values (>75%) and the relatively high Plasticity Indices (PI) (PI=LL– PL>20) indicated that the siltstone is moderate to highly expansive and therefore subject to shrink and swell cycles.
A further test was undertaken by subjecting sample to swell pressure test. The test confirmed that the siltstone material MH (Highly Plastic Silt) was really moderately expansive as it generated a confined swell pressure of 42.6 Kpa (890 psf).
This could have contributed to the disturbance and movements in the upper layer joints from shrinkage and expansion prior to the slide after saturation.
(6) Results of Field Borings
The Field Borings aided in characterizing the subsurface materials both within and outside the slide zone. It also indicated the depth and extent of disturbed materials of the slide zone and the groundwater conditions at the site after the slide.
In addition, it gave an indication of the Rock Quality through the percentage core recoveries and also through its Rock Quality Designation (RQD) values. The plot of the RQD values taken from the immediate area from 4 Boreholes and numerous core samples are shown in a Scatter Chart, Figure 4.0.
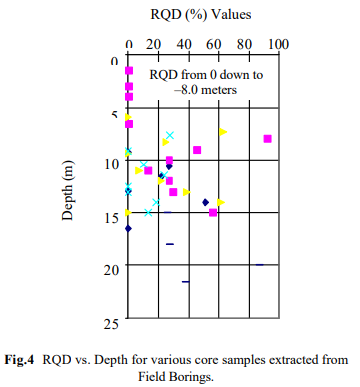
The RQD values as can be seen are relatively very low and indicate the highly fractured condition of the rock materials even in the undisturbed areas.
4. ENGINEERING STUDIES AND ANALYSES
(1) Determination of the Characteristic Rock Mass Strength (CRMS)
The shear strength of an intensely fractured rock is difficult to obtain as it would be difficult, if not impossible, to recreate or model the jointing and orientations of the rock under a full scale load test of the slope.
Wyllie1) stated that structures founded on Fractured Rock containing no distinct fracture surface on which sliding can take place, may still fail in shear if the shear strength of the rock mass is exceeded.
Because of the inherent difficulty of sampling and testing large samples of Fractured Rock, Wyllie proposed two empirical methods for determining the shear strength of the rock masses.
These two procedures are:
a) Back analyses of the failed slope setting the Factor of Safety equal to 1.0 and obtaining paired values of cm and Phi by varying the value of “cm“ and getting the corresponding Phi value. These paired values are then compared to historical records of slides that have been analyzed in the past using this same procedure.
b) Substitution into a formula using the Hoek Brown criterion in order to obtain values of cm and Phi by drawing a Mohr’s Circle diagram of the failure condition.
The engineering analyses procedures adopted in this report followed these two empirical procedures as recommended by Wyllie to obtain values that could be used for the ensuing stability analyses.
The engineering studies and analyses took into account the following information in order to reconstruct the slide mechanism:
a) Geometry of the slopes prior to the development of Cherry Hills as a Subdivision.
b) Post Development Slope Geometries and existing platform elevations prior to the slide.
c) Analyses of the slide geometry and locations of various landmark features.
d) Review of the air photos and their interpretation.
e) Recorded observations from various photographs of the site during the various field trips including location of displaced vegetation, houses, streets, etc.
f) Results of the Subsurface Soil Exploration, Test Pits and Laboratory Tests conducted.
From the foregoing, a composite picture could be formed and a fairly reliable model of slope geometry before the slide could be obtained.
(2) Slope Geometry Determination
Slope Geometry was obtained and verified from the results of the DTM and the GPS Surveys.
(3) Approximation of Rock Mass Strength
Closely or intensely jointed rocks behave more like particulate materials (soil like behavior) when disturbed and exhibit spoon shaped or stepped path slides at failure. Thus, it would not be correct to rely on the intact core strengths for a back analysis of the slide as it would invariably yield very high factors of safety.
In a similar vein, it would not be correct to expect that the intensely fractured Rock Masses would behave and move as huge intact blocks (Topples) as would normally be expected for less closely spaced jointed rocks.
Bieniawski2) developed an empirical approach to classifying highly jointed Rock Masses by the Rock Mass Rating (RMR). The RMR is a qualitative procedure to characterize fractured rocks. Based on this, the approximate RMR value for the Cherry Hill Rocks is as follows: RMR Sandstone = 30.
The RMR is in turn used by Hoek to quantify the shear strength of Fractured Rocks by yielding “m” and “S” values which could be applied to formulas to yield the characteristic shear strength of the Rock Mass based on a curvilinear failure envelope.
The “m” and “s” values as quantified by this procedure and used in this study are as follows:
Sandstone: m = 0.275 s = 0.00009
Siltstone: m = 0.183 s = 0.00009
(4) Back Analysis Procedure
To complement the empirical procedure of determining the characteristic strength of the Intensely Fractured Rock. Wyllie also proposed the use of Back Analysis of the Failure.
This procedure required carrying out a slope stability analysis with the factor of safety set at 1.0 (F.S. = 1.0) and using available information on the position of the failure surface, the ground water conditions at the time of failure, etc. This procedure however involves two unknowns, the Friction Angle “ϕ ” and the cohesion “c”. A number of stability analyses needed to be carried out with a range of cohesion values used to yield corresponding Friction Angle Values (ϕ ) by always setting F.S.=1.0.
The paired values (c, ϕ) obtained were then plotted in a chart of Cohesion vs. Friction Angle as shown in Fig. 5.0.
From this chart can be selected the most likely (c, ϕ ) value to be used.
While the “exact” (c, ϕ ) value at failure may not be known as there probably are other localized factors involved, the values obtained could give a fair approximation of the characteristic strength along the failure plane at impending slide.
5. SLOPE STABILITY ANALYSES
Slope stability analyses were performed using Slope/W, a proprietary Slope Stability Analysis Program.
SLOPE/W is a stability analysis program that uses the limit equilibrium theory to solve for the factor of safety of earth and rock slopes using various methods (Bishop, Janbu, Corps of Engineers, Morgenstern and Price, etc.)
The analyses consisted of runs at various stages in the predevelopment and development stages culminating in the final condition leading to the landslide.
(1) Identifying Critical Candidate Slope Failure Modes
The initial stability analyses performed generally used an assumed homogeneous soil mass and arbitrary strength parameters for the various layers, in order to initially identify possible failure modes and their locations. Subsequent analyses used the geologic model and layering sequences based on the Geologic Profiles.
(2) First Pass “Slope Stability Analyses”
A conventional “First Pass” slope stability analysis was conducted using the Characteristic Fractured Rock Mass Strength in order to define potential failure planes. This would aid in identifying where the critical conditions are, based on the slope geometries and characteristic Rock Mass Strength. Cohesion and Friction Angle (cm and ϕ m). The computer program was allowed to automatically search for these critical slip circles from which candidate locations as defined by low factors of safety were recorded. In addition, the search area was also concentrated on suspect critical areas in order to fully understand how the slide occurred and whether there was a single massive slide or a sequence of slides occurring one after the other within a few seconds.
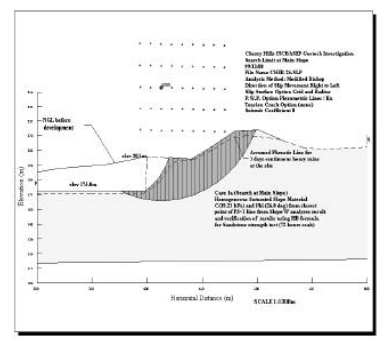
(3) Back Analyses Result
The procedure was conducted for both dry and saturated slope. (Figures 5 and 6)
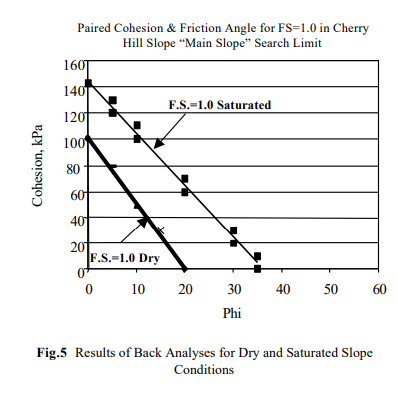
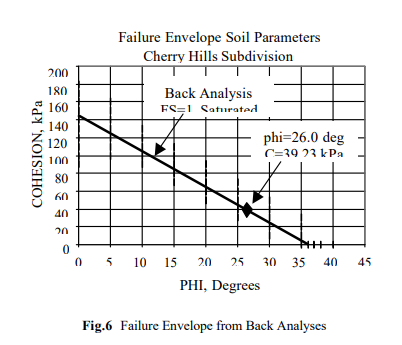
It is significant to emphasize what the above plot is telling us, which are:
a) For the same failure situation (FS=1.0) higher strength parameters (c & ϕ ) would need to be mobilized at saturated conditions to prevent failure. (Failure occurs at higher strengths)
b) Conversely, for dry conditions, lower strength parameters (c & ϕ ) would suffice to prevent a failure condition from occurring.
Thus, we can say that failure during high saturation and consequent pore pressure buildup was the most likely mode of failure.
The family of points show the possible combinations (paired values) of (c & f ) likely to cause failure. From within these paired values probably lie the characteristic Rock Mass Strength at time of failure.
(4) Final Slope Stability Analyses Results
The back analyses generally confirmed and agree with the actual slide geometry. The rotational component of the actual slide is clearly explained by the results of the slope stability analyses.
The results clearly indicate that the main failure mode is rotational and that the block sliding mode is less critical.
It also clearly shows that without saturation, failure would be remote.
6. VARIOUS INFLUENCES IMPACTING ON THE SLIDE INITIATION
(1) Climatic Effects
The Philippines encountered extremely severe prolonged draughts brought about by the El Niño Phenomenon. This extended period of extremely dry weather could have induced dessication and shrinkage cracking of the siltstone layers thus causing it to weaken.
Clay infillings on major joint systems could also have been induced to crack due to shrinkage from extremely dry weather.
Unfortunately, El Niño was immediately replaced by the unusually heavy rains which was totally the opposite of the El Niño. Periods of heavy constant rainfall were encountered in the month before the landslide event.
The two weeks preceding the landslide have seen the heaviest rainfall in the area in many years with the last 3 days immediately before the major landslide event the heaviest ever encountered.
The shrinkage caused by extremely dry weather induced cracking on the clay infilling of the joints, thus, further weakening the strength of the intensely jointed rock and causing some movements to occur.
The extremely heavy rainfall in turn induced the injection of water into these cracks and joints causing the following effects:
- Increased seepage and infiltration into the joints causing widespread lubrication of the Highly Fractured Rock Mass.
- Increased buoyancy or uplift effects, thus reducing effective stresses significantly.
- Increased water pressure induced swelling and expansion of the highly expansive siltstone layers causing heaving and disturbance of the intensely fractured rocks at some locations.
- Increased saturation induced weakening of the siltstone by as much as 35% based on simulated saturation conditions performed in the laboratory.
The very heavy rainfall in the weeks and days immediately preceding the Cherry Hills landslide event had contributed a critical part in the initiation of the slide. In fact, several other minor landslides in the areas surounding Cherry Hills were triggered days before the Cherry Hills landslide because of the heavy rainfall. Thus, clearly indicating the critical role of water saturation and buildup of pore pressure on the slide.
In this investigation, the only means to quantify or recognize the impact of water saturation in the analyses procedures were as folows:
- Strength degradation of the siltstone and sandstone layers by taking the mean strength at 72 hours saturation of the sandstone and siltstone layers as the intact Unconfined Compressive Strength qu (r) for use in the Hoek Brown formula and the back analyses of the slope stability.
- Taking into account the elevated phreatic line in the slope stability runs and thus also implicitly admitting the reduction in the effective stresses due to the elevated water table.
However, these effects in themselves were very significant as indicated by increased Factor of Safety at unsaturated (Dry) conditions and the very low Factors of Safety (Failure) resulting from the analyses simulating the above saturation conditions.
(2) Strength Degradation of Rocks
The results of the laboratory tests on the Siltstone layers obtained from intact block samples in the slide areas clearly established the Potentially Expansive nature of the siltstone layers as evidenced by:
- Very high Liquid Limit (LL) and Plasticity Indices (PI) averaging 80% and 22% respectively classifying therefore as MH.
- Generated Swell Pressure of approximately 802 psf in the siltstone sample subjected to confined swell pressure tests.
- Disintegration of cores when subjected to immersion after cooling from oven drying, crudely indicating or simulating the susceptibility to degradation due to drying and saturation effects.
- Significant strength degradation due to swelling and volume changes of siltstone core samples subjected to varying periods of water immersion (24, 48 & 72 hours).
Strength degradation of the siltstone layers was significant and movements in this layer due to shrinkage cracking and expansion due to prolonged water saturation could have affected or disrupted the insitu characteristic strength of the intensely fractured rock masses as to also have a contribution to the initiation of the landslide.
(3) Sandstone Layers
Strength degradation from saturation of the sandstones were not as pronounced as in the case of the siltstone cores. However, the presence of water at saturation levels clearly caused lubrication and reduction in effective stresses at the joints and weakening of the clay infillings as to have a contribution to the overall weakening of the intensely fractured rock masses.
(4) Intense Fracturing of the Rock Masses
The intense fracturing of the rock masses is visibly evident in the outcrops and the highly reduced sizes of the detrital materials in the slide.
The use of Triple Tube Core Barrels (Mazier Sampler) was resorted to in order to reduce the disturbance effects of the sampling procedure.
The intense fracturing of the rocks in Cherry Hills and surrounding areas is probably due to its proximity to the Binangonan Fault identified in the MGB Report. Faulting and associated movements near this fault could have caused this intense fracturing, reducing effective sizes to within a range of 150mm to 300mm. In the borings conducted at site, Rock Quality Designation (RQD) values were seldom above 25% indicative of Highly Fractured Rock condition even in the areas not involved in the slide.
As a result of this intense fracturing, the rock masses would tend to behave more as soil like materials rather than as intact coherent masses.
This is corroborated by the Post Slide Geometry which clearly shows the shape of the sliding mass. Most prominent is the spoon shaped configuration that is typical of Rotational Slides in soils.
(5) Creep and Elastic Heave
Cherry Hills Subdivision was sited on a massive cut from the crest of a mountain slope. The cuts average about 20 meters plus, based on reconstruction of the terrain prior to the excavations (NAMRIA Map) and its reconciliation with the Site Development Plan by using GPS spot elevations.
Such large volumes removed (overburden relief) could induce elastic heave and subsequent creep on the remaining subgrade.
Particularly, because the rocks are intensely fractured, the large overburden relief resulted in removal of confining stress which could have allowed elastic heave in the rock.
This was further aggravated by the presence of creep in the general subdivision platform area.
That creep was taking place is strongly supported by reported cracking and disturbances in the residential units experienced even several months before the slide. Interviews with residents clearly indicated that cracking of walls of houses and observed difficulties in closing windows and doors have been occurring months before the landslide.
(6) Unfavorable 8º Dip to SW
The Bedrock stratification pattern as confirmed by MGB Geologists indicated an unfavorable 8º Dip to the SW, of the bedding planes of the sedimentary rocks (Sandstone and Siltstone).
The slide originated from the Eastern slope and nearly followed the general SW direction of the Dip of the bedding planes.
The highly unfavorable situation probably also induced creep on the subdivision platform bedrock. Due to this unfavorable Dip towards Cherry Hills, the platform rocks were subjected to compressional forces even well ahead of the main landslide event thus causing creep and the consequent problems experienced in some of the houses in the subdivision. The presence of impermeable siltstone bands also probably contributed to the increased heaving because of the buildup in pore pressure underneath these impermeable bands.
This condition could further contribute to heaving and further destabilization in the critical hours before the landslide.
(7) Intervention by Man
Destabilization of the slopes in the Cherry hills area started soon after the large cuts made in the quarrying operations prior to subdivision development. The natural drainage pattern was altered.
Loss of lateral support of the slope and the existence of a steep nearly vertical slope face removed the buttressing effect of surrounding ground making the slopes inherently weakened and for joints to open up.
In addition, the natural gully at the back of the slope was suspected to have been filled up with spoils from the excavations which could have ponded water. However, this could not be verified.
The large unsupported cuts allowed the fractures to further open up later on to allow destabilizing factors to come in.
7. CONCLUSIONS FROM THE INVESTIGATION
(1) General
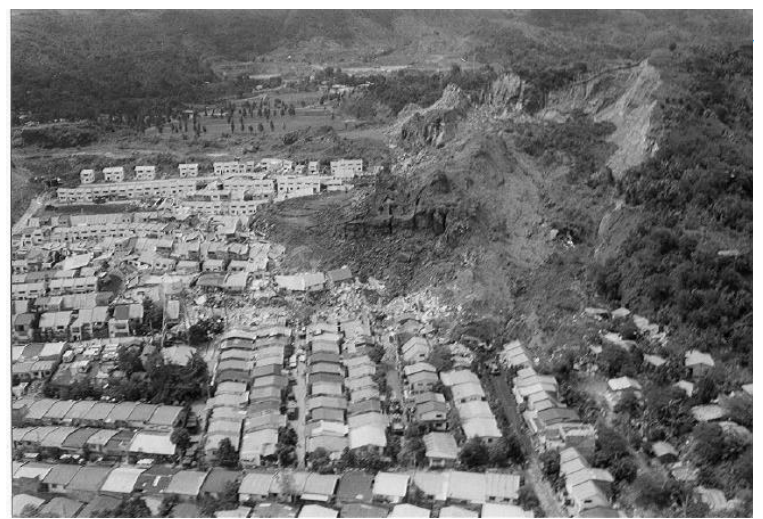
In the three days, August 1, 2 & 3 immediately preceding the landslide of August 3, 1999, record rainfall levels were experienced with the highest level of 500 mm occurring on August 2, 1999.
With the record level of rainfall, development of near full Hydrostatic Pore Pressure was possible. The diversion or blocking of intermittent and continuous surface streams by the landslide movement can add surface water (which originally flowed elsewhere) to the groundwater in the slide area.
These combined with the intensely fractured rocks and already weakened rocks to initiate the landslide.
The confluence of these seemingly unrelated and unfortunate events was therefore responsible for the landslide. Prolonged water saturation and subsequent pore pressure buildup was the final blow that triggered the landslide.
As borne out by the foregoing studies and findings, no single factor could have exclusively caused this landslide and it had to take several events and factors (geologic, environmental, human) to meet, in the slopes of Cherry Hills, to cause the slide.
(2) Summary of Findings
The findings of the Committee are as follows:
- Water saturation of the slope triggered the main slide although creep was already suspected to be occurring over time well before the main slide.
- The slide that occurred at Cherry Hills on the night of 03 August 1999 was a compound slide made up of a slump and a rotational component.
- The lateral and vertical deformation of several roadways near the NE quadrant of the subdivision was caused by a separate although associated mass slumping to the North.
- The rotational component towards the East to West direction was the main slide mechanism although this could have been preceeded or currently with a minor shallow slide at the toe of the slope.
- Corollary slides (Debris and Mudflow) occurred in the North and South gullies with the latter being more massive in terms of volume of earth and debris that were displaced.
- Creep which was ongoing very much earlier than the slide, contributed to the weakening of the subdivision platform shallow layer causing it to fold beyond the toe of the main slide in accordion like fashion when the main slide occurred.
- Not one, but several factors or influences coming together caused the landslide.
(3) Recommended Directions for Future Studies
The actual location of the slip plane needs to be further verified in addition to the limited Borings that were undertaken.
Seismic refraction equipment could be employed to map the subsurface and detect the stratigraphy and the shear zone in order to verify the conclusions made in this study.
REFERENCES
1) Wyllie, D. “Foundation on Rock”, page 64, 1st Ed Chapman Hall, London, 1992
2) Goodman, R.E. “Introduction to Rock Mechanics”, 2nd Ed., John Wiley & Sons, N.Y., N.Y. 1989, pp. 43 to 46.