Rammed Aggregate Piers / Geopiers: Strengthening Foundations with PGATECH Group
Geopiers, also known as Rammed Aggregate Piers (RAPs) or Rammed Aggregate Columns (RACs), are an innovative ground improvement technology used to enhance soil bearing capacity and control settlement. At PGATECH Group, we specialize in providing top-notch RAP solutions tailored to meet the specific needs of your construction projects. This article delves into the details of what RAPs are, their history, purpose, advantages, applications, desirability, and the problems they address.
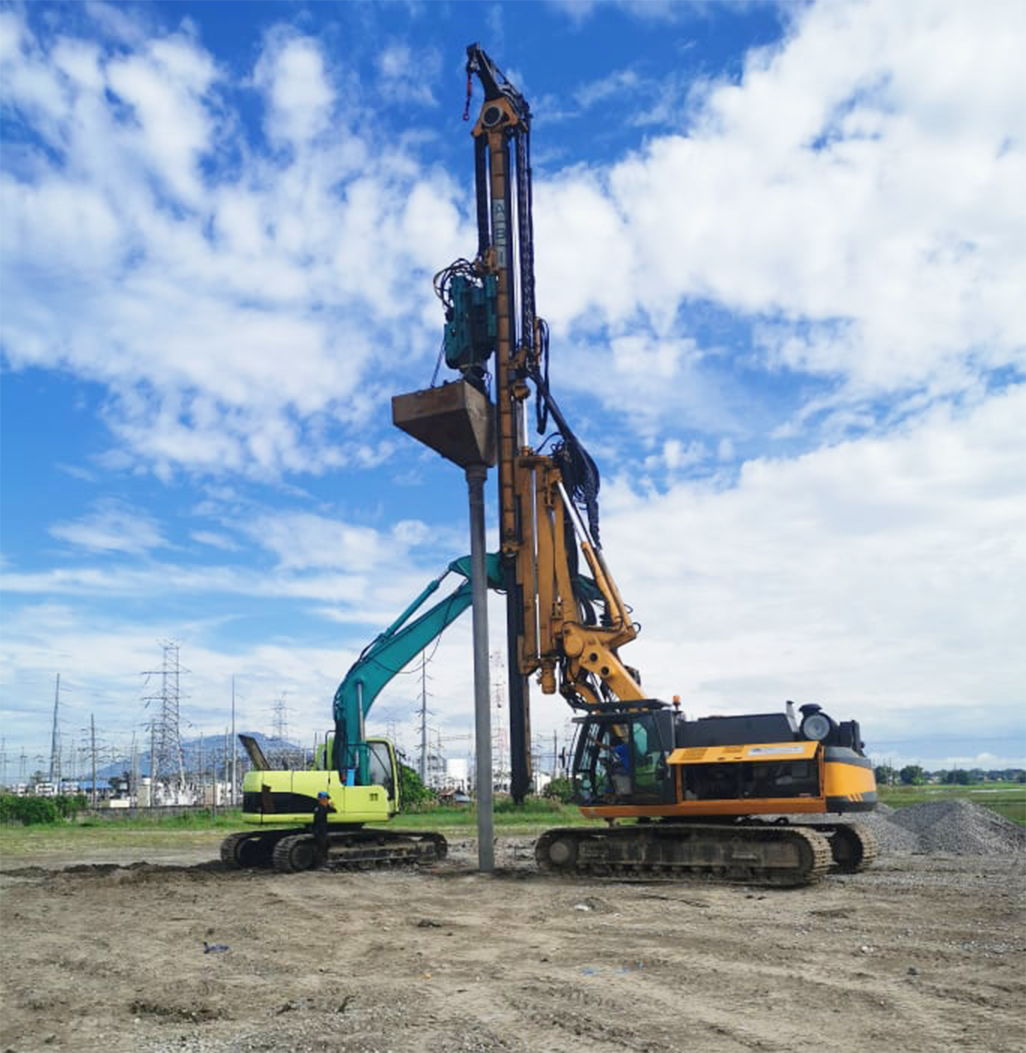
What are Rammed Aggregate Piers (RAPs) / Geopiers?
Geopiers, commonly referred to as RAPs (Rammed Aggregate Piers), are ground improvement elements created by densely compacting aggregate (crushed stone) into drilled holes in the ground. These piers or stiff columns reinforce weak soils, providing a robust foundation that can support heavy structural loads and reduce settlement.
History of Rammed Aggregate Piers (RAPs) / Geopiers
The concept of using aggregate to improve soil properties dates back to the early 20th century, but the modern technique of Rammed Aggregate Piers was developed in the early 1990s. The Geopier system was introduced as an alternative to deep foundations, offering a cost-effective and efficient method for ground improvement. Over the years, the technology has evolved, with advancements in installation techniques and equipment, making RAPs a reliable choice for many construction projects.
Rammed Aggregate Piers (RAPs) / Geopiers in the Philippines
Since October of 2000, the patented Rammed Aggregate Pier System has been used to support virtually all types of structures ranging from warehouses, tanks, embankments, low-rise to mid-rise buildings, MSE Walls, GRS Walls, and more.
Our clients from various industries have retained our services in their projects across the nation. Our team of experts and skilled personnel have also worked on government projects as Rammed Aggregate Columns have been recognized as a locally proven technology to support roadways and publicly-owned buildings.
What is the Function/Purpose of Rammed Aggregate Piers (RAPs) / Geopiers?
The primary purpose of RAPs/Geopiers is to improve the load-bearing capacity and reduce settlement of weak soils. The key functions include:
- Increasing Bearing Capacity: Enhances the strength of weak soils to support structural loads.
- Reducing Settlement: Controls and limits both total and differential settlement of structures.
- Improving Stability: Provides additional stability for foundations on problematic soils.
- Enhancing Soil Performance: Facilitates better load distribution and soil reinforcement.
What Foundation Systems Can Rammed Aggregate Piers (RAPs) / Geopiers Replace?
RAPs/Geopiers often serve as an effective alternative for more traditional and sometimes more expensive foundation solutions such as:
- Deep Foundations: Piles, drilled shafts, and caissons, which can be more costly and time-consuming to install.
- Over-Excavation and Replacement: Removing and replacing poor soil with engineered fill, which involves extensive excavation and material handling.
- Soil Stabilization Methods: Chemical grouting and soil mixing, which may not always be cost-effective or environmentally friendly.
Advantages of Rammed Aggregate Piers (RAPs) / Geopiers
RAPs/Geopiers offer several advantages, including:
- Cost-Effectiveness: Generally less expensive than deep foundation systems.
- Time Efficiency: Faster installation compared to traditional deep foundations.
- Flexibility: Suitable for a wide range of soil types and project conditions.
- Environmental Benefits: Uses natural materials (aggregate) and minimizes soil disturbance.
- Performance: Provides reliable improvement of soil bearing capacity and reduction of settlement.
Where are Rammed Aggregate Piers (RAPs) / Geopiers Applicable?
RAPs/Geopiers are applicable in various scenarios, such as:
- Commercial and Residential Buildings: Enhancing foundation support for buildings on weak soils.
- Industrial Facilities: Supporting heavy machinery and storage areas.
- Infrastructure Projects: Roads, highways, bridges, and railways.
- Embankments and Slopes: Stabilizing slopes and embankments to prevent landslides.
- Wind Turbines and Utility Structures: Providing a stable foundation for tall and heavy structures.
What Makes Rammed Aggregate Piers (RAPs) / Geopiers Desirable?
RAPs/Geopiers are highly desirable for several reasons:
- Efficiency: Quick installation process minimizes construction delays.
- Economic Savings: Lower overall project costs due to reduced material and labor requirements.
- Minimal Disruption: Less invasive installation compared to traditional deep foundations.
- Versatility: Adaptable to a variety of soil conditions and structural requirements.
- Sustainability: Environmentally friendly approach using natural aggregates.
Problems Addressed by Rammed Aggregate Piers (RAPs) / Geopiers
RAPs/Geopiers effectively address several common soil and foundation problems, including:
- Low Bearing Capacity: Enhances the ability of weak soils to support structural loads.
- Excessive Settlement: Controls and reduces settlement, ensuring long-term structural integrity.
- Soil Instability: Increases soil stability, preventing potential ground failure.
- Differential Settlement: Mitigates uneven settlement, which can cause structural damage.
- Construction Delays: Speeds up the foundation preparation process, allowing for timely project completion.
Conclusion
At PGATECH Group, we are committed to delivering high-quality Rammed Aggregate Pier / Geopier solutions that ensure the stability and durability of your construction projects. Our expertise and advanced techniques guarantee that even the most challenging soil conditions are effectively managed. For more information about our RAP/Geopier services, please contact us today.